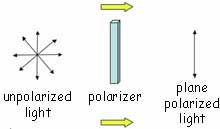
Fig. 1: Unpolarized light contains all E-vectors, while plane-polarized light contains a single E-vector direction. Click image for larger view.
Polarization Vision
Sönke Johnsen, PhD
Assistant Professor
Duke University
Tamara Frank, Ph.D.
Biological Oceanographer
Harbor Branch Oceanographic Institution
People with normal vision deal with two qualities of light every day – brightness and color. However, there’s a third quality of light that we humans cannot see, and that’s the direction of the electric vector (E-vector) of light, which is also known as the polarization of light.
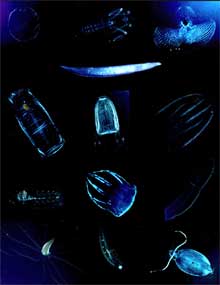
Fig. 2: A gallery of images of plankton viewed between two polarizers. In this particular setup, a normal transparent object would still be invisible. However, if the object, or animal, contains any birefringent substance, it will appear quite bright. Tissues such as muscle and tendon can be highly birefringent and are responsible for almost all the bright areas on these images. Click image for larger view and credits.
Unpolarized light contains all E-vectors, while plane-polarized light contains a single E-vector direction (Fig. 1). Completely polarized light is unusual in nature, but often, one plane of polarization will dominate. To our eyes, unpolarized and plane-polarized light looks the same. Crustaceans (shrimp, crabs) have eyes that are constructed very differently from ours, and because of these differences, they should be able to distinguish between different E-vectors. Visual pigment molecules themselves are polarization sensitive, because in order for light to be absorbed, the pigment molecule must be aligned with the E-vector of that particular photon of light. However, in vertebrate visual receptor cells (rods and cones), the visual pigment spins freely in the membranes of these receptors, making the receptor cell sensitive to all E-vector directions. In crustacean (and many other invertebrate) receptor cells, the visual pigment molecules are aligned in the receptor cell membrane, and this gives them greater sensitivity to a particular plane of polarization.
Behavioral experiments have demonstrated that a number species of near-surface animals, particularly shrimp and squid, see polarized light. In Deep-Scope 2004, we found that the ability to see polarized light greatly increases the ability to see transparent animals in the pelagic zone (figs. 2,3). This is because the tissues of many animals affect the polarization of the underwater light. This change, due to a property known as birefringence, is invisible to us, but quite obvious to anyone wearing Polaroid sunglasses or having polarization vision. Therefore, polarization vision may be a clever way for animals to find all those well-hidden transparent animals that we have talked about before.
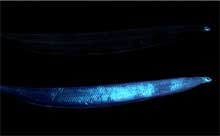
Fig. 3: Two images of the same leptocephalus eel larva. The top image is viewed under unpolarized, transmitted light. The bottom image is viewed under polarized, transmitted light by a camera with a polarizing filter. The increased visibility of the bottom image is due to the presence of birefringent muscle and connective tissue fibers. Click image for larger view and credits.
Polarization in animals and in the water
During our SCUBA dives, we will set up targets underwater and film them using a special camera that can see polarized light. The targets will be filmed over a range of distances and we will analyze the images in several ways. One method involves seeing how much more visible the target becomes when the background polarized light is removed (which makes the background darker, but leaves the target's brightness unchanged). We will also do this with live animals, since they have special optical properties that are different from the target's. We will feed the polarization images and video into an algorithm that was originally created to remove haze from atmospheric photos using polarization information and which shows great promise for increasing the contrast of underwater scenes as well.
In addition, we will measure the polarization of the water using an imaging system mounted on the Johnson-Sea-Link (JSL). This will measure the polarization of the light in the horizontal direction, which is important for fish trying to find their meals, and also the overhead polarization. The overhead polarization is gone at depth, but near the surface it is a distorted version of the polarization pattern of the sky. This pattern contains clues about the location of the sun, even if it is hidden in the clouds, and is used by honeybees, ants and some other animals to find their way back home after feeding.
We will also continue to measure the polarization properties of different animals that we collect. This information, along with the polarization properties of the water and the polarization-visual abilities of different animals, will give us a much better understanding of the uses of this enigmatic ability.
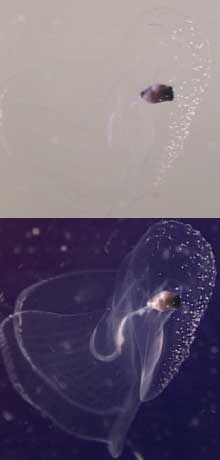
Sequential video frames through polarization video camera of marine zooplankton (mollusk). The increase in conspicuousness of the otherwise transparent creature in one frame may mimic what animals with polarization vision are capable of in such environments. The more 'invisible' frame is the way we see the animal. The potential for transparent-camouflage breaking using polarization vision is one of the questions we will examine during the DeepScope 2005 mission.
Determining which animals can see polarized light
In order
to test whether deep-sea species have polarization vision, we will be using
the same set-up that was described for the 2004 Deep Scope expedition (Vision
Essay). However,
this experiment is considerably more difficult than testing for color vision.
In the color vision experiments, white light is filtered to adjust color
and intensity, and then transmitted to the eye through a light guide. Changing
the plane of polarization is unfortunately not as easy as simply rotating
a polarizing filter in front of the light guide. The light guide,
being composed of thousands of individual fibers, depolarizes light,
so even if polarized light is sent through one end of the light guide,
the light hitting the eye would be completely depolarized. Placing
a rotating filter between the light guide and the eye results in almost
insurmountable technical difficulties, as the eye must remain inside a
Faraday cage (a metal cage used to block extraneous electrical noise that
would be picked up by the amplifier, such as from a computer) in complete
darkness during these experiments, and therefore physically manipulating
a filter to change the plane of polarization is simply not an option. However,
relatively new technology that is being used for underwater videography
by Justin Marshall and Tom Cronin will be adapted for use in these experiments.
Utilizing a 90º twisted nematic liquid crystal display, built by Doug
Bryant of the Liquid Crystal Institute, Kent State University, we will
be able to test the responses of the eye to two polarization planes, differing
by 90º. These
liquid crystal molecules are sandwiched between 2 pieces of glass, with
a polarizing filter on one surface. When no charge is applied to
the crystals, the light coming in through the polarized surface is rotated
90º (for
further information on twisted nematic LCs, see the discussion on http://electronics.howstuffworks.com/lcd1.htm ).
When a charge is applied to the crystals, all the molecules will line up
parallel to the surface of the glass, and the light is no longer rotated,
effectively giving us light whose primary E-vector orientation is 90º to
that in the uncharged state. Utilizing this technology ensures that the
position and angle of the light guide does not move when switching polarization
(as it might when reaching into the Faraday cage to attempt to manually
manipulate a polarizing filter), an absolutely critical requirement for
an experiment of this type.