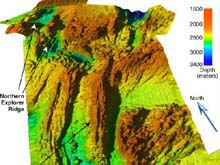
Three-dimensional image of Explorer Ridge viewed from the southwest looking northeast. Click image for larger view and a more detailed explanation. Click image for larger view and a more detailed explanation.
Seafloor Mapping
Bob Embley
Geophysicist
NOAA, Pacific Marine Environmental Laboratory
Producing maps of the seafloor has always been a particular challenge to humankind. The first primitive maps were rendered from successions of single soundings produced by lowering weighted lines into the water and noting when the tension on the line slackened, indicating the ocean floor. The depth was then measured by the amount of line paid out. These early maps gave only the most general picture of the ocean floor and only the larger features could be identified by looking for patterns in many such soundings. Most of these surveys were conducted to identify near shore hazards to shipping. Only in the late nineteenth century did expeditions begin to take large numbers of soundings in deep water.
A History of Seafloor Mapping
The first modern breakthrough in seafloor mapping came with the use of underwater sound projectors called sonar, which was first used during World War I. By the 1920s, the Coast and Geodetic Survey (the precursor to NOAA's Ocean Service) was using sonar to map deep water areas. The team of A. C. Veatch and P. A. Smith produced one of the first detailed maps of the ocean floor. This map showed that the canyons off the East Coast of the United States extended into very deep water. During World War II, advances in sonar and electronics led to much improved systems that provided precisely timed measurements of the seafloor at great water depths. These systems provided the databases to construct the first real maps of important features such as the deep-sea trenches and mid-ocean ridges. In 1957, the publication of Heezen and Tharp's physiographic map of the North Atlantic was the first map of the seafloor that allowed the general public to begin to visualize the ocean floor. These early maps, based on hundreds of thousands of hand-picked depths, provided the context for the plate tectonics revolution in the 1960s that finally gave science satisfying explanations for the formation of mid-ocean ridges, trenches, mountain ranges and the ring of fire around the Pacific.
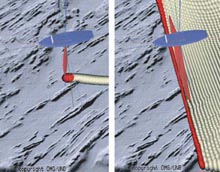
Comparing single (left) and multibeam (right) echo sounding of the seafloor. The multibeam method is preferred because it measures an entire area rather than a single line of seafloor. Image courtesy of University of New Brunswick. Click image for a larger view and a more detailed explanation.
Still, these systems only produced depth soundings immediately below the ship's tracks. To produce maps of the shape of the seafloor, one had to laboriously contour an area by connecting lines of equal depth together. Although the advent of digital computers in the 1960s provided much needed automation to the plotting of such data, the civilian scientific community was still using the same basic technology until the 1970s. In the 1960s, the U.S. Navy began using a new technology of multibeam sonar. Arrays of sonar projectors produced soundings not only along the track, but also for significant distances perpendicular to the ship's track. Instead of lines of soundings, these new multibeam systems produced a swath of soundings. Combined with automated contouring, the ability to make detailed, complete maps of large areas of the seafloor became available to the scientific community for the first time in the late 1970s after the Navy declassified the technology. Since these first multibeam systems, this technology has steadily improved, and modern systems can map swaths up to several times the water depth. Combined with positional information gained by the GPS navigation systems and advanced computer graphics, these systems now provide a whole new view of the seafloor.
Knowing the Seafloor
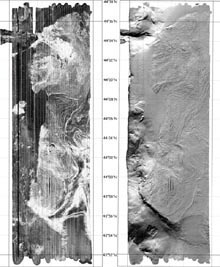
Heceta Bank Simrad EM 300 backscatter (left) and topography (right) on 10m grids. The backscatter image reveals seafloor physical properties and texture of the seabed. White areas depict cobble, boulder and sand areas. Black areas depict muddy areas. Click image for larger view and more detailed information.
The shape of the seafloor provides a critical part of our knowledge of the ocean. However, it is also critical to map changes in the composition of the seafloor. Whereas the depth can be measured using the timing of the signals going to and from the seafloor, it requires a precise measurement of the "strength" of the sonar return to make any judgments of the texture of the seafloor. For example, a sound pulse impinging on a mud seafloor will be mostly absorbed with only a small percentage returning to the receiver. However, a rock bottom will absorb very little sound and return most of it. In this way, modern seafloor survey systems include the measurement of relative strength of signal return as well as the depth.
The record produced from this consists of a pattern of variable strength signal returns that provide a picture of the seafloor's composition. An alternative method of mapping the texture of the seafloor is provided by a sidescan sonar system. These systems are towed on cables, and send and receive sound signals over a broad swath of seafloor. The sonar arrays are positioned so they project sound at low angles. The resulting record can detect very subtle features by the acoustic shadows that are cast. Depending on the pitch of the system (low pitch sound travels farthest), sidescan sonar can cover hundreds of meters to kilometers of seafloor.
Advantages and Challenges
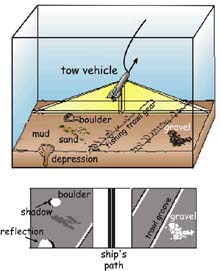
Schematic diagram of a sidescan sonar towed instrument insonifying the seafloor (top) and the sidescan data record created (bottom). Click image for larger view and a more detailed explantion.
Mapping the seafloor using a sonar array mounted on the hull of a research vessel has advantages. The vessel can travel at about 10 knots ( approx. 18.5 km/hr), it is very stable, and the data is generated in near real-time and can be processed into useable maps very quickly after it is acquired. However, as the water depth increases, the footprint of the beams generated by the sonar become larger, rather like the spreading out of the beam of a flashlight with distance. Since the sonar averages the echo return across each beam to generate a depth point, the spreading out of the beams decreases the resolution of the resulting map.
With increasing depth, an additional limitation surfaces. The higher frequency sound needed to generate very accurate maps increasingly loses energy (attenuates) as the amount of water it passes through increases.
The only practical method of making high-resolution maps of deep sites is to bring the sonar system closer to the seafloor. One method is to tow a fish containing the array. However, towing a fish at depth involves using long, heavy cables. The drag on cables effectively reduces the speed of the vessel to a few knots. A new method that is just becoming available is to mount the sonar on an autonomous underwater vehicle (AUV). During the Explorer Ridge expedition, engineers from Woods Hole Oceanographic Institution will, for the first time, mount a high-resolution multibeam sonar on the ABE. We anticipate that this will dramatically increase the exploration efficiency of the expedition by freeing up the R/V Thomas G. Thompson to conduct other operations while ABE surveys the seafloor.
Sign up for the Ocean Explorer E-mail Update List.